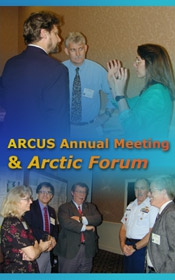
Overview
The ARCUS 13th Annual Meeting and Arctic Forum 2001 was held in Arlington, VA. In addition to highlighting important arctic research through the Arctic Forum,the ARCUS annual meeting serves to gather member institution representatives, the Board of Directors, and the staff of ARCUS together with other members of the arctic research community and key agency personnel and policy makers.
The Arctic Forum 2001 was chaired by Dr. John Hobbie, of the Marine Biological Laboratory, and included invited and contributed talks by arctic researchers representing diverse disciplines, projects, and programs. This year the Arctic Forum focused on interactions between the biological and physical systems. The four student recipients of the Fifth Annual ARCUS Award for Arctic Research Excellence presented their award-winning papers during the Forum as well.
2001 Arctic Forum Volume
The volume of abstracts from the 2001 Arctic Forum are available below in PDF format.
Poster and Presentation Abstracts for the ARCUS 13th Annual Meeting and Arctic Forum 2001
5/24/01 - 2/15/02Sheraton Crystal City Hotel, Arlington, Virginia, USA
To submit an abstract, click on the button below and follow these instructions as prompted:
- Provide the poster title and contact information for the first author,
- Add contact information for each of the poster authors in order,
- Select which author will be presenting the poster at the Arctic Forum or, if no authors will be at the Arctic Forum, provide contact information for the person we can contact regarding the poster;
- Paste in the text of the poster abstract,
- Answer the questions that follow so ARCUS can accommodate your needs.
Abstracts are listed in alphabetical order by first author's last name.
List of Abstracts
Benthic Community Composition and Biomass Distribution: Gulf of Alaska to the Canadian Archipelago
Arianne L. Balsom1, Jackie M. Grebmeier2, Lee W. Cooper3
1Department of Ecology and Evolutionary Biology, The University of Tennessee, 569 Dabney Hall, Knoxville, TN, 37996, USA, Phone +1.865.974.6160, Fax +1.865.974.3067, Merrow1 [at] aol.com
2Department of Ecology and Evolutionary Biology, The University of Tennessee, 569 Dabney Hall, Knoxville, TN, 37996, USA, Phone +1.865.974.2592, Fax +1.865.974.3067, jgrebmei [at] utk.edu
3Department of Ecology and Evolutionary Biology, The University of Tennessee, 569 Dabney Hall, Knoxville, TN, USA, Phone +1.865.974.2990, Fax +1.865.974.3067, lcooper1 [at] utk.edu
Benthic production and standing stock have been found in past studies to be variable within the Canadian Archipelago, and considered generally lower than in the Bering and Chukchi Seas. During the 2000 US-Canada biodiversity collaboration functioning as part of the St. Roch II "Voyage of Rediscovery" to the Northwest Passage, several "hot spots" of standing stock were encountered with biomass values rivaling sampled Bering and Chukchi Sea sites, with an extreme value of 81.95 gC/m2 at Hat Island off Requisite Channel in Queene Maude Gulf. Sampling within the Archipelago also demonstrated the other end of the biomass spectrum, finding a biomass value of 0.024 gC/m2 at Clifton Point off of Victoria Island, near Dolphin and Union Strait in Amundsen Gulf. Average biomass appears to be lowest in the Gulf of Alaska, reaching the highest measured average values in the Bering and Chukchi seas, and decreasing in the Beaufort Sea and into the Canadian Archipelago where the most extreme values of the cruise were found.
These results are preliminary due to continuing analysis of station samples, with numbers presented ranging from one to the four total replicates per station completed. Single grabs are presented for a preliminary spatial description. This spatial description indicates a possible trend of bivalve dominance in more southerly latitudes, Yoldia sp. dominant in the Gulf of Alaska, Nuculana radiata and Nucula belloti dominant in the Bering and Chukchi seas. The amphipod Ampelisca sp. and bivalve Macoma calcarea were dominant in the Bering Strait region. The polychaete Sternaspidae and amphipod Byblis sp. were dominant in the Beaufort Sea samples and entering the Canadian Archipelago. At Hat Island, bivalves were again dominant, consisting of Astartidae and Hiatellidae. The most northeasterly station (near Spence Bay, Nunavut) was largely dominated by sponge Porifera.
Reduced Growth in Alaskan White Spruce in the 20th Century from Temperature-induced Drought Stress
Valerie A. Barber1, Glenn P. Juday2, Bruce P. Finney3
1Institute of Marine Science and Forest Sciences Department, University of Alaska Fairbanks, PO Box 757220, Fairbanks, AK, 99775-7220, USA, Phone 907/474-7899, Fax 907/474-7204, barber [at] ims.alaska.edu
2Forest Sciences Department, University of Alaska Fairbanks, PO Box 757200, Fairbanks, AK, 99775-7200, USA, Phone 907/474-6717, Fax 907/474-7439, gjuday [at] lter.uaf.edu
3Institute of Marine Sciences, University of Alaska Fairbanks, PO Box 757200, Fairbanks, AK, 99775-7200, USA, Phone 907/474-7724, Fax 907/474-7204, finney [at] ims.uaf.edu
The extension of growing season at high northern latitudes seems increasingly clear from satellite observations of vegetation extent and duration 1,2. This extension is also thought to explain the observed increase in amplitude of seasonal variations in atmospheric CO2 concentration. Increased plant respiration and photosynthesis both correlate well with increases in temperature this century and are therefore the most probable link between vegetation and CO2 observations 3. From these observations 1,2, it has been suggested that increases in temperature have stimulated carbon uptake in high latitudes 1,2 and for the boreal forest system as a whole 4. Here we present multi-proxy tree-ring data (ring-width, maximum latewood density, and carbon-isotope composition) from 20 productive stands of white spruce in interior Alaska. The tree-ring records show a strong and consistent relationship over the past 90 years and indicate that, in contrast with earlier predictions, radial growth has decreased with increasing temperature. Our data show that temperature-induced drought stress has disproportionately affected the most rapidly growing white spruce, suggesting that under recent climate warming, drought may have been an important factor limiting carbon uptake in a significant portion of the North American boreal forest. If this limitation in growth due to drought stress is sustained, the future capacity of northern latitudes to sequester carbon may be less than currently expected.
Reduced Growth in Alaskan White Spruce in the 20th Century from Temperature-induced Drought Stress [Poster]
Valerie A. Barber1, Glenn P. Juday2, Bruce P. Finney3
1Institute of Marine Science and Forest Sciences Department, University of Alaska Fairbanks, PO Box 757220, Fairbanks, AK, 99775-7220, USA, Phone 907/474-7899, Fax 907/474-7204, barber [at] ims.alaska.edu
2Forest Sciences Department, University of Alaska Fairbanks, PO Box 7200, Fairbanks, AK, 99775-7200, USA, Phone 907/474-6717, Fax 907/474-7439, gjuday [at] lter.uaf.edu
3Institute of Marine Sciences, University of Alaska Fairbanks, PO Box 7200, Fairbanks, AK, 99775-7200, USA, Phone 907/474-7724, Fax 907/474-7204, finney [at] ims.uaf.edu
The extension of growing season at high northern latitudes seems increasingly clear from satellite observations of vegetation extent and duration 1,2. This extension is also thought to explain the observed increase in amplitude of seasonal variations in atmospheric CO2 concentration. Increased plant respiration and photosynthesis both correlate well with increases in temperature this century and are therefore the most probable link between vegetation and CO2 observations 3. From these observations 1,2, it has been suggested that increases in temperature have stimulated carbon uptake in high latitudes 1,2 and for the boreal forest system as a whole 4. Here we present multi-proxy tree-ring data (ring-width, maximum latewood density, and carbon-isotope composition) from 20 productive stands of white spruce in interior Alaska. The tree-ring records show a strong and consistent relationship over the past 90 years and indicate that, in contrast with earlier predictions, radial growth has decreased with increasing temperature. Our data show that temperature-induced drought stress has disproportionately affected the most rapidly growing white spruce, suggesting that under recent climate warming, drought may have been an important factor limiting carbon uptake in a significant portion of the North American boreal forest. If this limitation in growth due to drought stress is sustained, the future capacity of northern latitudes to sequester carbon may be less than currently expected.
Warming of the North Pacific Polar Front and its Possible Effect on the Bering Sea
Igor M. Belkin1, Richard Krishfield2, Susumi Honjo3
1Graduate School of Oceanography, University of Rhode Island, 215 South Ferry Road, Narragansett, RI, 02882, USA, Phone 401-874-6533, Fax 401-874-6728, ibelkin [at] gso.uri.edu
2Department of Geology and Geophysics, Woods Hole Oceanographic Institution, MS #23, Woods Hole, MA, 02543, USA, Phone 508-289-2849, Fax 508-457-2175, rkrishfield [at] whoi.edu
3Joint North Pacific Research Center, Woods Hole Oceanographic Institution, MS #23, Woods Hole, MA, 02543, USA, Phone 508-289-2521, Fax 508-457-2118, shonjo [at] whoi.edu
Over 200 meridional sections in the northern North Pacific have been analyzed, augmented with a number of surveys in the Gulf of Alaska, in order to map the Polar Front and study its long-term variability. The Polar Front was defined as the southern boundary of the "pure" Subarctic vertical structure that features a pronounced, very cold, subsurface temperature minimum (Tmin) underlain by a temperature maximum (Tmax). This boundary was typically associated with enhanced horizontal gradients of most parameters of vertical structure. These elevated gradients could be traced down to at least 1500-2000 m depth. The front extends non-zonally from 40°N off Japan to 57°N in the Gulf of Alaska where it retroflects and flows westward with the Alaskan Stream. The frontal parameters (first of all, temperature) change downstream and with time. The decadal variability of the front was studied along the 150°E, 170°E, 175.5°E, and 180°E sections, occupied annually. Most time series of the Tmin, Tmax, and T100 temperatures on both sides of the front revealed a dramatic long-term warming between 1978 and 1999. The magnitude of the Tmin warming is at least 1°C over 20 years (up to 2°C along 170°E). Since the Tmin is believed to be a remnant of wintertime convection, this warming signals a significant amelioration of the winter climate of the northern North Pacific over last two decades. The observed warming of the Polar Front and associated current is supposed to have been advected by the large-scale circulation to the Gulf of Alaska, Alaskan Stream and eventually, with the Alaskan Stream's northward branches, to the Bering Sea.
The SCICEX Database Project (SDP) - Developing An Interactive Arctic Environmental GIS
Paul A Bienhoff1, Jeffrey H. Smart2, Wayne Loschen3
1Undersea Systems Group, Strategic Submarine Dept, Johns Hopkins University Applied Physics Laboratory, Mailstop 24W445, 11100 Johns Hopkins Road, Laurel, MD, 20723-6099, Phone 240/228-4323, Fax 240/228-6864, paul.bienhoff [at] jhuapl.edu
2Submarine Technology Dept., JHUAPL
3Submarine Technology Dept., JHUAPL
The data collected during the SCICEX cruises from 1993-1999 is not readily accessible to other scientists. The SCICEX Database Project will correct this limitation, allowing future Arctic investigators to use the baseline data from the SCICEX investigations to focus and improve their investigations. The SDP will be an integrated database for viewing and analysis of environmental data collected during the SCICEX program. Though a good portion of the SCICEX data is available in digital form, much of it is not yet archived in the NSF-sponsored central data warehouse (ARCSS-DCC).
The Submarine Operational and Research Environmental Database (SOARED)
Johns Hopkins University Applied Physics Laboratory (JHUAPL) developed a database and display system to address a Navy requirement to make data collected by a submarine accessible to the crew of that submarine. In the course of the design of the Navy system (the FAST TACTical Integration Console, FAST TACTIC), a demonstration website was assembled. This demonstration website (http://wood.jhuapl.edu/soared/) is called the Submarine Operational and Research Environmental Database (SOARED) and uses data collected on SCICEX cruises plus data from other publicly accessible databases. The idea was to use data similar, if not identical, to the data collected by the SCICEX submarines, and show how that data could be retrieved and displayed using a simple graphical user interface (GUI). In addition, SOARED has some basic analytical features that allow data to be compared and evaluated statistically. Data are retrieved using queries generated with either "point and click" or "drag and drop" actions using a computer mouse.
The SCICEX Database Project (SDP)
SDP is intended to be a node linked to ARCSS-DCC, and archiving all SCICEX data collected to date as well as data collected on future submarine Arctic cruises. Subsequent to the development of the SOARED demonstration website, several of the scientists who have been involved in SCICEX suggested the fixed database could be useful for other scientists who could compare SCICEX data to data collected for their own work. This project addresses that suggestion. The presentation for the Arctic Forum will describe the project and include a demonstration of the existing relational database, displays and basic retrieval and analysis tools. A desired outcome of the presentation will be support for the concept, along with suggestions for other features that will enhance the scientific value of the SCICEX information.
The SCICEX Database Project (SDP) - Developing An Interactive Arctic Environmental GIS [Poster]
Paul A. Bienhoff1, Jeffrey H. Smart2, Wayne Loschen3
1Undersea Systems Group, Strategic Submarine Dept, Johns Hopkins University Applied Physics Laboratory, Mailstop 24W445, 11100 Johns Hopkins Road, Laurel, MD, 20723-6099, USA, Phone 240/228-4323, Fax 240/228-6864, paul.bienhoff [at] jhuapl.edu
2Submarine Technology Dept., JHUAPL, USA
3Submarine Technology Dept., JHUAPL, USA
The data collected during the SCICEX cruises from 1993-1999 is not readily accessible to other scientists. The SCICEX Database Project will correct this limitation, allowing future Arctic investigators to use the baseline data from the SCICEX investigations to focus and improve their investigations. The SDP will be an integrated database for viewing and analysis of environmental data collected during the SCICEX program. Though a good portion of the SCICEX data is available in digital form, much of it is not yet archived in the NSF-sponsored central data warehouse (ARCSS-DCC).
The Submarine Operational and Research Environmental Database (SOARED)
Johns Hopkins University Applied Physics Laboratory (JHUAPL) developed a database and display system to address a Navy requirement to make data collected by a submarine accessible to the crew of that submarine. In the course of the design of the Navy system (the FAST TACTical Integration Console, FAST TACTIC), a demonstration website was assembled. This demonstration website (http://wood.jhuapl.edu/soared/) is called the Submarine Operational and Research Environmental Database (SOARED) and uses data collected on SCICEX cruises plus data from other publicly accessible databases. The idea was to use data similar, if not identical, to the data collected by the SCICEX submarines, and show how that data could be retrieved and displayed using a simple graphical user interface (GUI). In addition, SOARED has some basic analytical features that allow data to be compared and evaluated statistically. Data are retrieved using queries generated with either "point and click" or "drag and drop" actions using a computer mouse.
The SCICEX Database Project (SDP)
SDP is intended to be a node linked to ARCSS-DCC, and archiving all SCICEX data collected to date as well as data collected on future submarine Arctic cruises. Subsequent to the development of the SOARED demonstration website, several of the scientists who have been involved in SCICEX suggested the fixed database could be useful for other scientists who could compare SCICEX data to data collected for their own work. This project addresses that suggestion. The presentation for the Arctic Forum will describe the project and include a demonstration of the existing relational database, displays and basic retrieval and analysis tools. A desired outcome of the presentation will be support for the concept, along with suggestions for other features that will enhance the scientific value of the SCICEX information.
Evolution of the Cold Halocline Layer in the Eurasian Basin of the Arctic Ocean
Timothy Boyd1, Michael Steele2, Robin Muench3, John Gunn4
1College of Oceanic & Atmospheric Sciences, Oregon State University, USA
2Polar Science Center, University of Washington, USA
3Earth & Space Research, USA
4Earth & Space Research, USA
Data from icebreaker and SCICEX submarine cruises of the 1990's document the retreat and subsequent recovery of the cold halocline layer (CHL) in the Eurasian Basin (EB) of the Arctic Ocean. The freshwater content from the ocean surface to the base of the halocline was determined for near-repeat transects extending from the Alpha Ridge to the Arctic Mid-Ocean Ridge. Comparison of summer and winter data is feasible because the existence of a residual signature of the winter mixed layer in summer profiles allows the upper ocean to be split into separate fresh water pools: seasonal ice melt, winter mixed layer, and winter halocline (WH). Significant interannual variations were observed in the freshwater content of the WH. The freshwater content in the WH decreased in the EB during the period 1991-1995 (the CHL retreat), and increased in the Amundsen and Makarov Basins during 1995-1999 (the CHL recovery). Over the period 1991-1999, the fresh water content in the WH increased in the northern Amundsen Basin. The disappearance of the CHL from the EB in 1995 has been attributed to an eastward shift (toward Bering Strait) of the injection point into the central basin of fresh water from the Russian shelves. IABP ice velocities and sea level pressure fields suggest that the reappearance of the CHL in 1999 was due to a westward shift in the injection point.
El'gygytgyn Crater Lake in NE Siberia: Deep Sediment Fill Promises 3.6 million year Arctic Paleoclimate Record
Julie Brigham-Grette1, Olga Yu Glushkova2, Pavel Minyuk3, Matt A. Nolan4, Melles Martin5, Nowaczyk Norbert6, Neissen Frank7, Bernd Wagner8, Conrad Kopsch9, Celeste A. Cosby10, Vladimir Smirnov11, Michael Apfelbaum12, Grisha Federov13, Anatoly Lozhkin14, Pat Anderson15, Marina Cherapanova16
1Geosciences, University of Massachusetts, Amherst, MA, 01003, USA, Phone 413-545-4840, Fax 413-545-1200, juliebg [at] geo.umass.edu
2NorthEast Interdisciplinary Scientific Research Institute, Russian Academy of Sciences, 16 Portovaya street, Magadan, Russia, smirnov [at] neisri.magadan.ru
3NorthEast Interdisciplinary Scientific Research Institute, Russian Academy of Sciences, 16 Portovaya street, Magadan, Russia, minyuk [at] neisri.magadan.ru
4Water and Environmental Research Center, University of Alaska Fairbanks, Fairbanks, AK, 99775, USA, fnman [at] uaf.edu
5Institute for Geophysics and Geolog, University Leipzig, Talstrasse 35, Leipzig , D-04103, Germany, melles [at] rz.uni-leipzig.ed
6Laboratory for Paleo- and Rock Magnetism, GeoForschungsZentrum Potsdam, Telegrafenberg Haus C, Potsdam, D-14473, Germany, nowa [at] gfz-potsdam.de
7Alfred Wegener Institute, Columbus St, Bremerhaven, 27515, Germany, fneissen [at] awi-bremerhaven.de
8Alfred Wegener Institute, Telegrafenburg 843, Potsdam, D-14473, Germany, bwagner [at] awi-potsdam.de
9Alfred Wegener Institute, Potsdam, D-14473, Germany, ckopsch [at] awi-potsdam.de
10Department of Geosciences, University of Massachuse, Amherst, MA, 01003, USA, ccosby [at] geo.umass.edu
11NorthEast Interdisciplinary Scientific Research Institute, 16 Portovaya, Magadan, Russia, smirnov [at] neisri.magadan.ru
12Department of Geosciences, University of Massachusetts, Amherst, MA, 01003, USA, michaela [at] geo.umass.edu
13Arctic & Antarctic Research Institute, St. Petersburg, Russia, dolshiyanov [at] aari.nw.ru
14NorthEast Interdisciplinary Scientific Research Institute, Magadan, Russia, lozhkin [at] neisri.magadan.ru
15Quaternary Research Center, University of Washington, Seattle, WA, USA, pata [at] u.washington.edu
16Institute of Biology and Soil Sciences, Far East Branch of the Russian Academy of Sciences, Vladivostok, Russia, pushkar [at] fastmail.vladivostok.ru
In 1998 we recovered what is now the longest lacustrine paleoclimate record in the Arctic at 400 ka from El'gygytgyn Lake, in Northeast Siberia. Because this lake lies inside a non-glaciated impact crater created 3.6 million years ago, we have the potential to recover a straightforward climate record representative of the western Arctic dating back to the middle Pliocene. Core analyses thus far have shown, for example, that boreal treeline migrated north and then south of the crater during the last interglacial, demonstrating a sensitivity to land/climate interactions. Field studies this year provided data important to the interpretation of the 1998 core and an understanding of the modern processes, including modern limnology, geomorphology, coring, stream and lake hydrology, local meteorology, and a 2-fold seismic program including airgun and 3.5 kHz high-resolution profiles.
Initial field results from the seismic data indicate that the total sediment fill in the basin is more than of 370 m draped over a small central impact cone in this crater nearly 18 km in diameter. These data also indicate the best sites for a deep drilling program. Fragments of paleoshorelines at elevations roughly 45 m, 18 m, 8 to 12 m and 6 m, especially around the east and south shores, indicate that lake level has been higher in the past; the highest levels probably occupied early in the lake history but difficult to date. Alluvial fans with slopes of 3-4 degrees around more than half of the lake margin consist primarily of alluvium and soliflucted colluvium at the current surface; emergent lacustrine shelf sediments occur in only a few sections. Nearly half of the lake basin lacks any wide shelf, including large areas fronting larger alluvial fan complexes. Modern beaches around the lake are coarse with high storm berms related to waves and ice shoving created by the long fetch and strong regional winds. Detailed studies of the sedimentology, modern and down core studies of the pollen, diatoms, and geochemistry are about to be published.
El'gygytgyn Lake is ice covered roughly 9 months of the year, becoming ice-free usually by early-mid July. Once ice-free, our measurements show the lake mixes completely by wind stress maintaining a temperature of 2 to 3 degrees C; there is no significant thermocline. Lake temperature, lake level, and meteorology equipment have been installed at the lake to provide us with several years of in situ data. Initial meteorology data suggest that the local climate of El'gygytgyn is representative of regional synoptic climatology indicating that the paleoclimate record from this lake is a proxy of broad scale western arctic environmental change. Analysis of the 1998 core confirms this by showing teleconnections between various paleoclimate proxies with the GISP core, which contains a time-series less than half as long as the El'gygytgyn core.
Sedimentology and clay mineralogy of the upper 6 meters of the core representing the entire late Pleistocene show distinct changes in illite-smectite and chlorite percentages consistent with cold vs warmer intervals in the core. The data show that clay mineralogy and sedimentology, in addition to magnetic susceptibility (MS), TOC, pollen, and diatoms provide and important means for interpreting past change. The sedimentology also supports our notion that MS is a direct proxy for climate reflecting changes in the lake ice cover and oxygenation of the bottom waters as dictated by paleotemperature.
Fifty-year Record of Coastal Erosion along Elson Lagoon, Barrow, Alaska
Jerry Brown1, Torre Jorgenson2, Matt Macander3
1International Permafrost Association, P.O. Box 7, Woods Hole, MA, 02543 , USA, Phone (508) 457-4982, Fax (508) 457-4982, jerrybrown [at] igc.org
2ABR, Inc., PO Box 80410, Fairbanks, AK, 99708, USA, Phone 907-455-6777, tjorgenson [at] abrinc.com
3ABR, Inc., PO Box 80410, Fairbanks, AK, 99708, USA
Erosion rates of coastlines dominated by ice-rich permafrost are among the highest on Earth. Although erosion is limited to 3-4 months of ice-free water, rates may exceed 10 m/yr. Erosion and accretion of northern coasts are the focus of the new international project Arctic Coastal Dynamics (ACD) http://www.awi-potsdam.de/www-pot/geo/acd.html. Major focus is on the contribution of coastal erosion to the sediment budget of the inner continental shelf, with emphasis on sources and fate of organic carbon.
The U.S. Beaufort Sea coast provides important sites for evaluating long-term coastal changes and the potential contributions of coastal sediments to cross-shelf transport. Observational sites are proposed at representative coastal segments including the six North Slope Borough villages, ANWR and Prudhoe Bay. The Elson Lagoon coast near Barrow, Alaska, provides an excellent location for long-term monitoring of rates of erosion and sediment yields and their fate. The study is part of the 7,466 acres Barrow Environmental Observatory (BEO) of arctic tundra that was permanently set aside for research in 1992 by the Ukpeagvik Iñupiat Corporation (UIC). Bluff elevations in the study area range between 2 and 4 m and are dominated by polygonal ground consisting of ice-rich, fine-grained sediments, reworked peats, and ice wedges.
Using 1948/49 and 1962/64 aerial photography, Lewellen measured erosion rates from Brant Point eastward approximately 30 km to Dease Inlet. We updated the time series of coastline changes using sequential aerial and satellite imagery from 1949, 1962-64, 1979, 1997, and 2000 for a 10-km long section of the same coastline. Aerial photographs were rectified to a high-resolution (1 m) IKONOS satellite image base map. Rectification accuracy (relative to the 2000 satellite image) ranged from 0.69 to 2.56 m among periods. When compared to erosion rates measured over a long period, the effect of measurement error is relatively small (e.g., up to 0.1 m/yr for a 20-yr period). Coastlines were digitized on these images and used to determine the area of land lost for each period.
Photogrammetric analysis reveals high spatial variation in rates of coastal erosion. In a broad-scale comparison of four contiguous sections (2.0–3.4 km long) along the coast, erosion rates ranged from 0.7 m/yr to 3.0 m/yr for the period 1979 to 2000, with an overall erosion rate of 1.3 m/yr. (total loss of 16-58 meters in the 21 years). The several sections with low rates are more protected by a shallow offshore shoal. The section with greatest erosion has a larger fetch and water depths up to 3 meters or more; accounting for the increase in rates. Loss of land due to erosion on a 2.5-km section over the 51-year period was 110 m or 21.3 acres. The August 10–14, 2000 storm with its 1.5-m surge along the adjacent Chukchi Sea coast resulted in 1.1 m of erosion in the northern section of coast along Elson Lagoon.
Exchange of Greenhouse Gases Between Arctic Terrestrial Ecosystems and the Atmosphere
Torben R. Christensen1
1Climate Impacts Group, Centre for Geobiosphere Studies, Lund University, C/O Dept of Ecology, Lund, 22362, Sweden, Phone +46462223743, Fax +46462224423, torben.christensen [at] planteco.lu.se
Application of Geographical Information System (GIS) Techniques to Assessing Benthic Biological Change in The Bering Sea
Jackie L. Clement1, Lee W. Cooper2, Jackie M. Grebmeier3
1Department of Ecology and Evolutionary Biology, The University of Tennessee, 569 Dabney Hall, Knoxville, TN, 37996, USA, Phone +1.865.974.6160, Fax +1.865.974.3067, jlc [at] utk.edu
2Department of Ecology and Evolutionary Biology, The University of Tennessee, 569 Dabney Hall, Knoxville, TN, 37996, USA, Phone +1.865.974.2990, Fax +1.865.974.3067, lcooper1 [at] utk.edu
3Department of Ecology and Evolutionary Biology, The University of Tennessee, 569 Dabney Hall, Knoxville, TN, 37996, USA, Phone +1.865.974.2592, Fax +1.865.974.3067, jgrebmei [at] utk.edu
In the northern Bering Sea, there exists a region of historically high benthic biomass and abundance. This region is located on shallow continental shelves southwest of St. Lawrence Island. During winter, a polynya forms just south of the island while surrounding water is covered by more than 90 percent ice. From the early 1990's to the late 1990's a decline in bivalve abundance and biomass is apparent. Diving sea ducks, which feed on bivalves, have also undergone a dramatic decline. Primary production, benthic biomass and abundance, bivalve size classes, percent ice cover, ice extent, sediment grain sizing, Beryllium-7, conductivity, temperature, and depth have all been measured in this region over the previous decade.
Using GIS (Geographic Information System) software, I will try to determine which factors are important in causing this decline. Data from multiple cruises will be placed in a GIS for further analysis using two software products, ArcView 3.2 and ArcInfo 8. Using the same projection and basemap will allow for the standardization of various years. Because station data only gives information for one point, interpolation between stations is necessary to produce a continuous view of each parameter.
Many important questions arise in regard to this benthic decline. What caused the decline? Is it a cyclic event? In order to understand biological change in the Bering Sea, one must face the challenge of differentiating between interannual and seasonal processes. Benthic macroinvertebrates are not only affected by multiple processes within the sediments, but are also greatly affected by processes in the overlying water column, such as sedimentation. With the help of GIS technology I plan to uncover patterns and create a model for the northwest Bering Sea benthos.
Ecosystem Change in the Northern Bering Sea
Lee W. Cooper1, Jacqueline M. Grebmeier2
1Department of Ecology and Evolutionary Biology, University of Tennessee, 569 Dabney Hall, Knoxville , TN, 37996, USA, Phone 865/974-2990, Fax 865/974-3067, lcooper1 [at] utk.edu
2Department of Ecology and Evolutionary Biology, University of Tennessee, 569 Dabney Hall, Knoxville, TN, 37996, USA, Phone 865/974-2592, Fax 865/974-3067, jgrebmei [at] utk.edu
Mapping Thermal and Hydrological Conditions beneath a Polythermal Glacier with Radio-echo Sounding
Luke Copland1, Martin Sharp2
1Department of Earth and Atmospheric Sciences, University of Alberta, Edmonton, AB, T6G 2E3, Canada, Phone 780/492-4156, Fax 780/492-7598, luke.copland [at] ualberta.ca
2Department of Earth and Atmospheric Sciences, University of Alberta
Spatially contiguous patterns in residual bed reflection power (BRPr) are used to map the thermal and hydrological conditions at the base of a high Arctic polythermal glacier. Residual bed reflection power represents the difference between measured and predicted bed reflection powers, once the influence of dielectric loss with ice depth has been accounted for. Areas with crevassing and other englacial features were removed from analysis since large internal reflections may reduce the power that reaches the glacier bed. Most surveys were made in the spring, while the snowpack was dry, to minimize the influence of variable coupling between the antenna and glacier surface. Correlation plots show that bed slope does not have a significant effect on BRPr.
Based on our findings, several conclusions can be made about the thermal structure of the glacier. Positive BRPr and the presence of an internal reflecting horizon over the glacier terminus suggest a warm basal layer in this region. In comparison, positive BRPr and the absence of an internal reflector in overdeepened and valley bottom areas in the upper ablation zone suggest that the pressure melting point is only reached at the glacier bed. Finally, negative GRPr and the absence of an internal reflector in all other regions are indicative of cold ice. Within the positive BRPr regions, variability in BRPr shows patterns similar to subglacial hydrological reconstructions and observations. Maximum BRPr values occur in areas where drainage is predicted, and an elongated area of high BRPr occurs directly upglacier from an artesian fountain which brought large volumes of turbid meltwater to the glacier surface. These observations imply that water at the glacier bed is a major control on BRPr. This is probably because water has a higher dielectric contrast with ice than any other subglacial material.
Relationships between Short-term Velocity Variations and the Subglacial Hydrology of a Polythermal Glacier [Poster]
Luke Copland1, Peter Nienow2, Martin Sharp3
1Earth & Atmospheric Sciences, University of Alberta, Edmonton, AB, T6G 2E3, Canada, Phone 780/492-4156, Fax 780/492-7598, luke.copland [at] ualberta.ca
2Geography & Topographic Science, University of Glasgow, Glasgow, G12 8QQ, UK
3Earth and Atmospheric Sciences, University of Alberta, Edmonton, AB, T6E 2E5, Canada
To investigate the relationships between ice flow dynamics and the characteristics and seasonal evolution of the subglacial drainage system of a polythermal glacier, surface velocities were determined for successive two day periods in summer 1998 and 1999 at John Evans Glacier, Ellesmere Island. Two distinct high ice motion events were recorded in 1999, and one in 1998. These events occurred during periods of rapidly increasing meltwater input to the glacier bed: (i) at the start of the summer melt season, and (ii) as air temperatures rapidly rose after a mid-summer cold spell (in 1999).
The magnitude of velocity increases during these events was non-uniform, with highest increases above regions of the glacier bed where subglacial drainage is predicted. Early season events were focused close to the glacier snout, where an artesian fountain that occurred in 1998 indicates that basal water pressures reached at least 120% of ice overburden pressure. The mid-season event was focused at the top of the terminus region above an area where vertical velocities suggest closure of drainage passages during the preceding cold spell. These observations suggest that the high motion events were due to enhanced basal motion driven by high basal water pressures that are localised along subglacial drainage pathways.
The Arctic System Science Data Coordination Center (ADCC)
Rudy J. Dichtl1, C. McNeave2
1National Snow and Ice Data Center, University of Colorado, Campus Box 449, Boulder, CO, 80309-0449, USA, Phone 303/492-5532, dichtl [at] kryos.colorado.edu
2National Snow and Ice Data Center, University of Colorado, Campus Box 449, Boulder, CO, 80309-0449, USA, Phone 303/492-1390, mcneave [at] kryos.colorado.edu
Archeological Investigations: 49-PET-408 (On-Your-Knees Cave), Southeast Alaska
E. James Dixon1
1Institute of Arctic and Alpine Research, University of Colorado, Campus Box 450, Boulder, CO, 80309-0450, USA, Phone 303-735-7802, Fax 303-492-6388, jdixon [at] spot.colorado.edu
to be submitted
Preliminary Results From Three Lakes From Ellesmere Island, Nunavut: Sawtooth, Tuborg and Murray
Pierre Francus1, Whit Patridge 2, Ray Bradley3, Bruce Finney4, Doug Hardy 5, Ted Lewis6, Bianca Perren7, Joe Stoner8, Mark Abbott9
1Geosciences, University of massachusetts, Amherst, MA, 01003-9297, USA, Phone 1-413-545-0659, Fax 1-413-545-1200, francus [at] geo.umass.edu
2Geosciences, University of Massachusetts, Amherst, MA, 01003-9297, USA, patridge [at] geo.umass.edu
3Geosciences, University of Massachusetts, Amherst, MA, 01003-9297, USA, rbradley [at] geo.umass.edu
4Institute of Marine Science, University of Alaska Fairbanks, Fairbanks, AK, 99775, USA, finney [at] ims.uaf.edu
5Geosciences, University of Massachusetts, Amherst, MA, 01003-9297, USA, dhardy [at] geo.umass.edu
6Geosciences, University of Massachusetts, Amherst, MD, 01003-9297, USA, lewist [at] geo.umass.edu
7Geosciences, University of Massachusetts, Amherst, MA, 01003-9297, USA, perren [at] geo.umass.edu
8Geology, University of California, Davis, Davis, CA, 95616, USA, stoner [at] geology.ucdavis.edu
9Geosciences, University of Massachusetts, Amherst, MA, 01003-9297, USA, mabbott [at] geo.umass.edu
This project intends to utilize the analysis of cores from 3 annually laminated lakes to provide high resolution late-Holocene environmental records and to study the temporal and spatial patterns of environmental change.
South Sawtooth Lake, Ellesmere Island (79°20' N, 83°51' W), is an oligotrophic lake located at the southwestern part of Fosheim Peninsula. We have focus our efforts on the 82 meter deep distal basin, which is almost completely meromictic. The basin is protected from the direct influence of turbidites and sedimentation is only due to settling. The basin contains annual clastic laminations: coarse and fine silt sediment during the snow melting season, followed by the settling of clays during the ice covered winter season. We produced a continuously varved 4.53 meter long sequence combining short a Glew core and a long vibracore. According to the varve count and 210Pb dates, this record spans the last 2550 yrs. The sequence has been studied for diatoms, organic content, paleomagnetism, geochemistry, and sedimentary facies. Using an image analysis technique of thin-sections (Francus, 1998), we produced multivariate and quantified data for each varve for the upper section of the sequence. The data obtained on each varve of the uppermost section of the cores have been compared with meteorological and climatological data, e.g. temperature, snow melt, wind, and stream discharge. For the last 33 years, snow-melt intensity correlates well with the median grain-size measured for each annual lamination. Summer rain events are also recorded as thin non-erosive beds of sand. This model is then used to infer environmental conditions with annual resolution from downcore laminae. We produced a reconstruction of the summer rain intensity based on the occurrence of sand layers. We discuss these fluctuations on the entire sequence, their cyclicity and compare them to the paleomagnetic record, other proxies retrieved so far, and other records in the Arctic.
Lake Tuborg, located at 80°58' N, 75°32' W on central Ellesmere Island, Nunavut, is 12 km long and consists of an 85 m deep proximal basin and a 140 m deep distal basin. Discharge into the northern side of the lake is dominated by snowmelt, whereas the adjacent Agassiz Ice Cap on the southern side of the lake controls the influx of freshwater. Long cores, taken above the chemocline of the proximal basin, have revealed varves that detail 300 years of high energy hydrological discharge events (Smith 1997). Smith demonstrated that the high resolution sediment records, formed in the sediment basin that is dominated by glacial meltwater input, correlate with summer melt layers from Agassiz Ice Cap cores (Fisher and Koerner, 1995) and Eureka MSC temperature records. For this project, a series of short cores were retrieved in May of 2000 along a transect that extends from the northern to the southern side of the deep, distal basin. The sediment cores, all taken below the chemocline, contain laminated sediments and are being analyzed using an image analysis technique. Image analysis of the laminated microstructures will be used to distinguish between the varves that formed on the nival side from those on the glacial side of the lake basin. This information will be used to discern the paleoclimatological conditions that formed varves in the deepest part of the basin, where sediment input originates from both sources.
Murray Lake is located on the eastern coast of Ellesmere Island at 81°20' N, 69°30' W. The lake is approximately 5 km2 and 50 m deep and lies 60 m above Archer Fjord. Runoff into the lake is dominated by nival melt from the west, spill over from the Upper Murray Lake to the north and a combination of nival and glacial melt from the Simmons and Murray Ice Caps to the east. Two short cores were retrieved from the northern basin in June of 2000 in 45 m of water. The short cores contain 1100 years of sedimentation and contain few disturbances or turbidites.
This material is based upon work supported by the National Science Foundation under grant no. 9708071
Polar Research -- A Window to Earth's Past, Present, and Future
Submitted by David D. Friscic for NSF, OPP1
1Office of Polar Programs , National Science Foundation , 4201 Wilson Boulevard , Arlington, VA, 22230, USA, Phone 703-292-8031 , Fax 703-292-9080 , dfriscic [at] nsf.gov
Polar Research: Study of the polar regions helps us see earth in innovative and unexpected ways. Knowledge of the region is vital to understanding responses of Earth's systems to natural and manmade changes. Polar environments interact with global processes in complex, significant ways. The regions are sensitive monitors of ongoing changes, but also encompass the historical record of similar changes in the past. The National Science Foundation's Office of Polar Programs supports research in the Arctic and the Antarctic, ranging from single investigator projects to multi-investigator, mulit-institutional, and international programs.
ARCTIC RESEARCH
- Human Dimensions of Climate Change: Changes in the marine environment and the impacts of overfishing affect coastal communities worldwide. Researchers supported by the OPP's Arctic Social Sciences and Arctic Systems Science programs tracked selected communities in Newfoundland, Greenland and Norway and analyzed the resulting data to describe how the communities are adapting to change. Demographic, economic, and sociological patterns are emerging.
- Atmospheric Studies: The arctic tundra historically has been considered a carbon sink. Recent research suggests that increased temperatures and snowfall during the winter and spring may now be causing the release of small amounts of carbon into the atmosphere. Because of this evidence San Diego State University investigators expanded earlier studies of the processes controlling carbon dioxide emission and uptake from a single watershed to a circumpolar scale.
- Ozone Depletion: TOPSE (Tropospheric Ozone Production about the Spring Equinox). Scientists from the Universities of Washington, Virginia, and New Hampshire did airborne weekly studies of an annual springtime rise in lower-atmosphere ozone levels and measured, for the first time, various chemicals that could shed light on ozone production, atmospheric cleansing, and pollution transport in the northern latitudes. The peculiar chemistry of the arctic spring is key to understanding ozone and pollution processes.
- Arctic Seafloor Mapping: Using the newly developed SCAMP(Seafloor Characterization and Mapping Pods), invesitgators from Tulane University, University of Hawaii's Hawaii Mapping and Research Group, and Lamont-Doherty Earth Observatory (LDEO) mapped previously uncharted areas of the Arcic Ocean Floor. SCAMP, developed at LDEO, provided the first high-resolution bathymetric map of the Gakkel Ridge, gravity-anomaly data, narrow-beam bathymetry, and "chirp" sub-bottom profiler data for inclusion in an arctic bathymetric map.
- Marine ecosystems: In 1997 and 1998 unusual weather significantly changed the hydrography and water circulation in the eastern Bering Sea. In July 1997 working along the inner front of the southeastern Bering Sea,investigators from Universities of California (Irvine), Alaska, and New England found a major ecosystem shift in the Barents Sea when a large coccolithophore bloom (E.huxleyi) developed for the first time. During the ensuing fall, nearly 10 percent of the region's 16 million short-tailed shearwaters, which migrate yearly from Australia, died, a likely result of the E. huxleyi bloom.
SUPPORT FOR ARCTIC RESEARCH
Approximately 600 scientists conduct more than 130 OPP-sponsored projects each year in the Arctic. In most cases, OPP's Arctic Research Support and Logistics program funds logistical support. About 50 percent of the researchers work in Alaska, with the remainder working in the Arctic Ocean, Canada, Greenland, Russia, and Scandinavia.
Facilities:
- Northwest of Barrow, Alaska, the Barrow Environmental Observatory (BEO),managed by the Barrow Arctic Science Consortium (BASC), is a permanent research site for tundra ecology, permafrost, climate, and arctic environmental studies.
- The 25-year-old Toolik Field Station is the only NSF Long-Term Ecological Research site in the Arctic.
- Summit Camp, Greenland, near the summit of the Greenland Ice Sheet, operates seasonally and in 1997-98 operated during the winter for the first time. A skiway is maintained for landings by LC-130 and other aircraft. OPP's arctic logistics contractor, VECO Polar Resources, supports research at the camp through Thule Air Base or the Kangerlaussuaq International Science Support Facility in West Greenland operated by the Danish Polar Center.
Ships:
- Besides two polar-class icebreakers "Polar Sea" and "Polar Star", which provide limited support for marine science studies, the U.S. Coast Guard operates the 420-foot icebreaker "USCG Healy".
- Through agreement with the U.S. Navy , U.S. scientists have recently used nuclear submarines (in the SCICEX program). The submarines can access any part of the Arctic Ocean permitted by water depth and can spend 40 to 60 days annually collecting data.
- "Alpha Helix", part of the U.S. academic research fleet, undertakes oceanographic research in high latitudes. Primary support comes through NSF's Division of Ocean Sciences, with joint support from OPP.
Air Support:
The 109th Airlift Wing of the New York Air National Guard (ANG) operates ski-equipped C-130 (LC-130) airplanes to support Summit Camp,Greenland, and to transport researchers to field projects throughout Greenland. Chartered helicopters, Twin Otter airplanes, and other aircraft provide access to remote field sites and are used to extend the geographic range around central research stations and camps. Helicopters are used where travel over land, snow or ocean is dangerous, slow, or damaging to the environment.
Data Telemetry From Arctic Locations Via the Iridium Satellite System
Robert H. Heinmiller1, Susan K. Kubany2, Tonya M. Taylor3
1Omnet, Inc., PO Box 1285, Staunton, VA, 24402, USA, Phone 540.885.5800, Fax 540.885.0132, R.Heinmiller [at] Omnet.org
2Omnet, Inc., PO Box 1285, Staunton, VA, 24402, USA, Phone 540.885.5800, Fax 540.885.0132, S.Kubany [at] Omnet.org
3Omnet, Inc., PO Box 1285, Staunton, VA, 24402, USA, Phone 540.885.5800, Fax 540.885.0132, T.Taylor.Tonya [at] Omnet.org
Migration from the Russian North During the Transition Period
Timothy E. Heleniak1
1Development Economics, The World Bank, MC2-209, 1818 H St., N.W., Washington, DC, 20904, USA, Phone 202-473-2540, Fax 202-522-3339, theleniak [at] worldbank.org
A majority of Russia's crucial raw materials are located in its Northern periphery. During the Soviet period, there was a unique set of development practices that existed to exploit the resources of the Northern regions. These included financial and other incentives for people to move to and work in the North and the construction of large urban agglomerations in the region. The result was that Russia had a much more densely populated North than other countries with comparable high-latitude regions.
One unintended consequence of Russia's transition to a market economy has been a massive out-migration from the Northern periphery. From the 16 regions defined as North in this study, over 10 percent of the population has migrated out since 1989. At the extreme are several Northern regions where over half the population has left during this period. Those leaving tended to be younger and more highly educated in general those more able to do so. Many older and less able persons are left in the North without the resources to be able to leave. The major causes of this out-migration have been price liberalization; fiscal decentralization; and a shift in Russia's approach to the development of its Arctic and sub-Arctic regions.
The study examines patterns of migration in the Russian North during the transition period beginning with a brief history of the settlement of the Russian North. Data are presented showing the composition of the Northern population prior to transition, followed by a description of the levels, direction, age-sex composition, educational, occupational characteristics, and mechanisms of Northern migration trends. The final section attempts to determine the possible future levels of migration from the North that can be expected.
The Chemical Composition of Meltwaters Draining a High Arctic Glacier
Karen E. Heppenstall1, Martin J. Sharp2
1Department of Earth and Atmospheric Sciences, University of Alberta, 1-26 Earth Sciences Building, University of Alberta, Edmonton, AB, T6G 2E3, Canada, Fax (780) 492 7598, keh [at] ualberta.ca
2Department of Earth and Atmospheric Sciences, University of Alberta, 1-26 Earth Sciences Building, University of Alberta, Edmonton, AB, T6G 2E3, Canada
This study aims to evaluate the nature and evolution of the hydrological system of polythermal John Evans Glacier, Ellesmere Island (80°N, 74°W) using natural tracers present in meltwater. Bedrock underying the glacier consists primarily of carbonates (limestone and dolostone) and evaporites (mainly gypsum/anhydrite).To detect changes in meltwater chemistry that occurred as water passed through the glacier, hydrochemical data were collected from snow, ice marginal lakes, supraglacial and subglacial streams on John Evans Glacier during the summer of 2000. Electrical conductivity (EC), pH and total alkalinity were measured in the field, and concentrations of major ions were determined by ion chromatography. Suspended sediment concentrations were also determined. To identify the major sources of variability in water chemistry, Principal Component Analysis (PCA) was performed on each of the datasets. Three phases of drainage system development were identified from these analyses. Phase I followed the seasonal onset of subglacial drainage, and was characterised by high, but steadily decreasing, concentrations of all major ions, and by trace amounts of Li+. Comparison of supraglacial and subglacial values indicates that most solute in waters draining from the glacier was derived by subglacial weathering. The chemistry of these waters suggest they have been stored beneath the glacier over winter, and illustrate progressive dilution by the influx of the new season's melt. During Phase II, solute concentrations stabilised at lower levels, and total alkalinity levels were similar in subglacial waters and waters draining into the supraglacial system from ice marginal lakes. Sulphate levels were, however, still considerably higher in the subglacial waters than in surface runoff. This suggests a marked reduction in the residence time of water at the glacier bed, such that solute acquisition became dominated by dissolution of evaporites rather than carbonates. Phase III was characterised by a sharp increase in discharge and SSC, and by increasing concentrations of Ca2+, Sr2+, HCO3- and SO42- in the subglacial water. Mg2+ concentrations were, however, unaffected, suggesting that solute acquisition was now from calcite and gypsum, but not from dolomite. The pCO2 of subglacial waters dropped sharply at this time, suggesting that much of the solute was derived from rapid in-channel dissolution of suspended sediment, that was mobilised by rising discharges in what was now an efficient channelised drainage system.
The Influence of Hydrologic Change on Arctic Biology
John E. Hobbie1, Bruce J. Peterson2
1The Ecosystems Center, Marine Biological Laboratory, 7 MBL Street, Woods Hole, MA, USA, Phone 508/289-7470, Fax 508/457-1548, jhobbie [at] mbl.edu
2The Ecosystems Center, Marine Biological Laboratory, Woods Hole, MA, USA, Phone 508/289-7484, Fax 508/457-1548, peterson [at] mbl.edu
Scenarios of climate change in the Arctic include changes in precipitation amounts--usually precipitation increases as the air warms. But these increases do not necessarily lead to wetter soils or more river runoff because evapotranspiration from land and plants will also increase with arctic warming. Also, the effect of warming on hydrology will depend on the site in question. For example, the Tanana Flats in Alaska are slightly marshy now but with melting of the permafrost the land surface will subside and the Flats could be inundated throughout the year.
Changes in freshwater amount and fluxes will affect arctic organisms in many ways. Microbial activity in soils may decrease if soils become wetter and anaerobic conditions become more frequent. This would lead to increased carbon storage in soils. On the other hand, a decrease in soil moisture may lead to increased decomposition rates and long-term decreases in carbon storage in arctic soils. Plants, at the same time, will increase in mass from the nitrogen released by the increased decomposition of soil organic matter under drier conditions. At a different scale, fish in streams will be strongly affected by the amount of stream flow; in the Kuparuk River, for example, arctic grayling grow faster in summers with high discharge rates than in low discharge summers. The key link between the change in hydrology and biologic response in this case may be the amount of insect drift in the stream, the principal source of food for the grayling. Finally, at even bigger scales, a pulse in freshwater discharge into the Arctic Ocean is thought to have contributed to the formation of "The Great Salinity Anomaly". The arrival of this cold, low-salinity arctic surface water off Iceland in the 1970s and 1980s resulted in a drastic reduction in phytoplanktonic production and eventually in lower survival of fish larvae. After some years, a reduction in the annual fishery catch of herring was seen, a fish vital to the Iceland economy.
Humans and the Arctic Environment: Changing Roles, Changing Research
Henry P. Huntington1
1Huntington Consulting, 23834 The Clearing Drive, Eagle River, AK, 99577, USA, Phone 907-696-3564, Fax 907-696-3565, hph [at] alaska.net
For most of the time that humans have inhabited Arctic regions, they have depended on locally available resources and the impacts of their activities have been similarly local. Only in the past few centuries or less have more distant forces--resource exploitation, large-scale immigration, pollution--begun to act on the Arctic and its inhabitants. For the most part, consideration of the role of humans in the Arctic environment has focused on the mechanistic connections between human activities and environmental conditions and vice versa. By the late 20th century, however, the simple connections and feedbacks between Arctic inhabitants and their surroundings had been altered by modernization. Some aspects are relatively straightforward, such as the way that imported foods have reduced reliance on local wildlife populations. Other aspects are more complex, such as the degree to which indigenous groups have acquired the political strength to affect policies that shape today's Arctic. The role in the Arctic of "humans as political animals" is an intriguing challenge for 21st century research.
Oil Biodegradation in the Arctic Marine Polluted Environments: Comparative Seasonal Study
Vladimir V. Il'inskii1, Michail N. Semenenko2
1Chair of Hydrobiology, Biological Faculty, Moscow State Lomonosov University, Biol. Faculty MSU, Vorob'evi gori, 119899 Moscow, Russia, Izmaylovskii bulvar 34-25, Moscow 105043, Russia, Moscow, Russia, Phone 965-03-54, Fax 939-01-26, ilinskiivladimir [at] mtu-net.ru
2Chair of Radiochemistry, Chemical Faculty, Moscow State Lomonosov University, Chem. Faculty MSU, Vorob'evi gori, 119899 Moscow, Russia, Moscow, Russia, Phone 939-38-45
Microbiological and hydrochemical investigations was done on the hydrological cuts coast-sea and river-sea near port Amderma (Kara Sea) in August and in the inner part of Kandalaksha Bay not far from Kandalaksha city (White Sea) in summer (August) and late winter (March). Hydrocarbons concentration in the coastal regions near Amderma varied in water from 37 to 260 µg/l, and in the inner part of Kandalaksha bay - from 10 up to 110 µg/l (was measured only in March). High concentration of hydrocarbons was observed in the marine sediments in the inner part of Kandalaksha Bay - from 23 to 600 µg/g in summer and from 8 to 265 µg/g in winter. Other abiotic parameters in coastal Kara Sea region: water temperature - 2,0 - 5,5 C; phosphate and nitrate concentrations - 0,02 - 0,23 µg-at/l and <0,03 - 0,11 µg-at/l correspondingly. In the inner part of Kandalaksha Bay water temperature varied from 4,5 to 15 C in summer and from 2,0 to -2,0 C - in winter; phosphate and nitrate concentrations in winter varied from 0,17 to 0,69 µg-at/l and from 0,66 to 2,26 µg/l correspondingly. Natural microbial mineralization potential (NMMPoct) of hydrocarbons (velocity of their oxidation by microorganisms to CO2 and H2O), measured in the samples of water at in situ temperature with using of 14C-octadecane as a substrate, in the coastal Kara Sea waters varied from 13 to 29 ng•l-1•h-1. In the inner part of Kandalaksha bay in summer NMMPoct values varied from 42 to 90 ng•l-1•h-1 and reduced during ice cover period not more than in two times. In some winter water samples near the same NMMPoct values as in summer was observed. The reasons for weak seasonal variations of the potential hydrocarbon-oxidizing microbial activity are discussed and the human pollution in couple with high active mesophilic and psychrophilic microbial populations may be one of the main.
Physical Changes in the Arctic and Their Affect on Animal Behavior and the Subsistence Activities of Arctic Indigenous Peoples
Charles H. Johnson1
1Alaska Nanuuq Commission, PO Box 924 - Belmont Point, Nome, AK, 99762, USA, Phone 907/443-5044, Fax 907/443-5060, cjohnson [at] nook.net
The scientific community has shown that decreases in ice thickness and cover, changes in participation, weather patters, ocean currents, etc. prove that global warming is a very real thing. Indigenous peoples also have reported changes in the environment and changes in the behavior and migration patterns of animals. They have reported absence of species and new species coming in. These changes have had and will have a greater impact on the subsistence activities that are the backbone of the culture of indigenous peoples in the Arctic.
Contributions of Traditional Knowledge to Understanding Climate Change in the Canadian Arctic
Dyanna Jolly [Riedlinger]1, Fikret Berkes2
1Natural Resources Institute, University of Manitoba, Winnipeg, MB R3T 2N2, (current) Burnham School Road, RD 5, Christchurch, 8021, New Zealand, Phone +64 (3) 347.680, dyjolly [at] pop.ihug.co.nz
2Natural Resources Institute, University of Manitoba, Natural Resources Institute, University of Manitoba, Winnipeg, MB, R3T 2N2, Canada
Despite much scientific research, a considerable amount of uncertainty exists concerning the rate and the extent of climate change in the Arctic, and how change will affect regional climatic processes and northern ecosystems. Can an expanded scope of knowledge and inquiry augment understandings of climate change in the North? The extensive use of the land and the coastal ocean in Inuit communities provides a unique source of local environmental expertise that is guided by generations of experience. Environmental change associated with variations in weather and climate has not gone unnoticed by communities that are experiencing change firsthand. Little research has been done to explore the contributions of traditional knowledge to climate change research. Based in part on a collaborative research project in Sachs Harbour, Western Canadian Arctic, we discuss five areas in which traditional knowledge may complement scientific approaches to understanding climate change in the Canadian Arctic. These are the use of traditional knowledge (i) as local scale expertise; (ii) as a source of climate history and baseline data; (iii) in formulating research questions and hypotheses; (iv) as insight into impacts and adaptation in Arctic communities; and (v) for long term, community-based monitoring. These five areas of potential convergence provide a conceptual framework for bridging the gap between traditional knowledge and Western science, in the context of climate change research.
Inuvialuit Observations of Climate Change: Bridging Traditional Knowledge and Science in the Canadian Arctic [Poster]
Dyanna Jolly [Riedlinger]1, Fikret Berkes2, Community of Sachs Harbour3
1Natural Resources Institute, University of Manitoba, Winnipeg, MB R3T 2N2, (current) Burnham School Road, RD 5, Christchurch, 8021, New Zealand, Phone +64 (3) 347.680, dyjolly [at] ihug.co.nz
2Natural Resources Institute, University of Manitoba, Natural Resources Institute, University of Manitoba, Winnipeg, Manitoba, R3T 2N2, Canada, berkes [at] ms.umanitoba.ca
3Sachs Harbour, NWT, Canada
The Arctic is considered by many to be the "canary" of climate change, and has become a focal point for climate change research. However, considerable uncertainty remains concerning the rate and extent of change, and the impact on northern ecosystems. Inuvialuit in the Canadian Arctic possess a substantial body of knowledge and expertise related to climate and climate change. In recent years, communities such as Sachs Harbour are experiencing changes that they consider beyond the range of normal or expected variability. Community assessments of change are based on cumulative knowledge of local trends, patterns and processes, derived from generations of reliance on the land. Can these community assessments, based on local observations and traditional knowledge, enrich and expand understandings of Arctic climate change? Here, we describe five convergence areas that can provide a framework for using Inuvialuit knowledge and western science together to understand climate change in the Canadian Arctic.
Concurrent Density Dependence and Independence in Populations of Arctic Ground Squirrels
Tim J. Karels1, Rudy Boonstra2
1Division of Life Sciences, University of Toronto at Scarborough, 1265 Military Trail, Scarborough, ON, M1C 1A4, Canada, Phone 604/822-5942, karels [at] zoology.ubc.ca
2Division of Life Sciences, University of Toronto at Scarborough, 1265 Military trail, Scarborough, ON, M1C 1A4, Canada, Phone 416/287-7419, Fax 416/287-7642, boonstra [at] scar.utoronto.ca
No population increases without limit. The processes that prevent this can operate in either a density-dependent way (acting with increasing severity to increase mortality rates or decrease reproductive rates as density increases), a density-independent way, or in both ways simultaneously1-3. However, ecologists disagree for two main reasons about the relative roles and influences that density-dependent and density-independent processes have in determining population size4,5. First, empirical studies showing both processes operating simultaneously are rare6. Second, time series analyses of long-term census data sometimes overestimate dependence7,8. By using a density-perturbation experiment9-12 on arctic ground squirrels, we show concurrent density-dependent and density-independent declines in weaning rates, followed by density-dependent declines in overwinter survival during hibernation. These two processes result in strong, density-dependent convergence of experimentally increased populations to those of control populations that had been at low, stable levels.
Coastal Effects on Cloud Forcing in Arctic Polynyas
Erica L. Key1, Peter J. Minnett2
1Meteorology and Physical Oceanography, Rosenstiel School of Marine and Atmospheric Science, University of Miami, 4600 Rickenbacker Causeway, Miami, 33149-1098, USA, Phone 305/361-4657, Fax 305/361-4622, ekey [at] rsmas.miami.edu
2Meteorology and Physical Oceanography, Rosenstiel School of Marine and Atmospheric Science, University of Miami, 4600 Rickenbacker Causeway, Miami, FL, 33149-1098, USA, Phone 305/361-4104, Fax 305/361-4622, pminnett [at] rsmas.miami.edu
Cloud cover in the vicinity of polynyas and leads remains an important but highly variable parameter in the surface heat budget. Although generally serving as a negative feedback for solar radiation in summer months, clouds are intimately linked to atmospheric water vapor and latent heat flux at the surface. Further complicating the polynya dynamic is the presence of topography in the form of mountains, islands, or coastal plains, which modify the boundary layer flow and influence cloud formation. Using data collected over four different polynyas within the Western Arctic, the influence of multiple and single coastlines, islands, and open-ocean topographies on cloud cover and open water is quantified.
Preliminary calculations of net cloud effect in the North Water (NOW) Polynya and on surrounding coastlines indicate that the coastal and marine environments are strongly decoupled by the high relief of Ellesmere Island. Due to orographic forcing, clouds over the coastal site tend to be cumuliform or cirriform in nature. The natural distribution of these cloud types tends to attenuate incoming solar radiation without contributing significantly to the downward longwave component associated with lower-level clouds. During periods of stratus cover, added longwave input emitted by cloud bases offsets, at least in part, the reduced incoming shortwave radiation. However, the occurrences of low-level stratus are too few and too short in duration to reverse the general negative trend of net cloud effect associated with increased cloud cover in late Spring.
Comparatively, the offshore environment is populated by stratus and cumuliform clouds that cover a larger percentage of the sky. Fluctuations in the net cloud effect in the marine environment are more sensitive to the amount and distribution of clouds than type, as forcing effects among the three major cloud types are of comparable magnitude (Hanafin and Minnett, 2001).
Analyses of the other polynyas follow the same logic, comparing cloud time series and radiative elements with onshore data. These studies also determine the dependence of cloud forcing on solar zenith angle on a case-by-case basis. Relative errors in the clear-sky shortwave parameterization will better define the range of tuning coefficients necessary for successful application in both marine and land-based environments.
Cycles in the Forest: Mammals, Mycophagy, and Mycorrhizae.
Gary A. Laursen1, Rodney D. Seppelt2, Maggie Hallam3
1Institute of Arctic Biology, University of Alaska Fairbanks, Department of Biology and Wildlife, 305A Bunnell, Fairbanks, AK, 99775-6100, USA, Phone 907/474 6295, Fax 907/474 6185, ffgal [at] mail.uaf.edu
2Biology, Australian Antarctic Division, Channel Highway, Kingston, Tasmania, 7050, Australia, Phone 011 61 (03) 62 , Fax 011 61 (03) 62 , rod.seppelt [at] antdiv.gov.au
3Biology and Wildlife, University of Alaska Fairbanks, 316 Bunnell, Fairbanks, AK, 99775-6100, USA, Phone 907/474 6295 , Fax 907/474 6185, mhallam [at] alaska.net
Depiction of the forest cycle involves symbiotic white spruce, Picea glauca (Moench) Voss var. albertiana (S. Brown) Sarg., as a host to numerous epigeous mycorrhizal fungi and 12 hypogeous ectomycorrhizal ascomycetes (4 sp.) and basidiomycetes (8 sp.), parasitic spruce broom rust fungi, Chrysomyxa arcotostaphyli Diet., and small mycophagous mammals, principally three species: Northern Flying (Glaucomys sabrinus) and Red (Tamiasciurus hudsonicus) squirrels, and the Tundra Redback vole (Clethrionomys rutilus).
Healthy white spruce live in mutualistic symbiosis with mycorrhizal fungi. Fungal mycelia engulf spruce root tips. Mycelia are much finer than either roots or root hairs and the spruce benefit by this increased surface area for the absorption of labile nutrients and water from otherwise nutrient poor soils. Spruce also gain physical protection for its root tips engulfed by the mycelium. This "gloved casing" provides a barrier from other microorganisms seeking to invade roots. Mycorrhizal fungi also produce antimicrobial compounds that deter competition from other fungi. In turn, the fruitbodies of mycorrhizal fungi benefit from a supply of sugars and amino acids from its host roots. Spruce may even be growing in more northern boreal forest locations where they would otherwise not persist without the advantages of the mycorrhizal relationship. Concomitantly, the mycorrhizal fungi would not be present without the spruce.
Parasitic fungi, and specifically Spruce Broom Rust (Chrysomyxa arcotostaphyli), occur abundantly in the boreal forests of interior and southeast Alaska. It is here that the range of spruce and kinnikinnick or mealberry (Arctostaphylos uva-ursi (L.) Spreng. var. uva-ursi ) coincide. Germinating rust spores on the spruce result is a perennial systemic infection. Fungus produced auxins cause prolific branching of the spruce and the limb mass is called a Witches Broom. Regions of the spruce other than the broom continue to grow normally. Fruiting of the rust fungus occurs on the broom's needles causing an orange coloration. In the fall, needles are shed and the broom appears as a mass of dead twigs. Northern Flying and Red squirrels take advantage of these dense branch clumps. Squirrels "hollow out" brooms, raise their young in these hollows, and then cache limb-dried epigeous and hypogeous mycorrhizal fungi for their winter food supply.
Trees ultimately die from repeated attack by parasitic rust fungi, insects, and mechanical damage. A host of decomposer heart and root rot fungi begin the process toward eventual felling of the dead trees. On the forest floor, the fallen spruce continues to play a critical role in mammal mycophagy and the mycorrhizal cycle by providing convenient raised walkways as "highways for travel" over the forest floor for the animals which in turn leave spore-rich feces as they go. Some spores even require this "right of passage" through a rodent's gut as a necessary precursor to germination, which completes this forest cycle.
This complex biological system is dynamically balanced through the physical environment where any changes will be reflected in the biology. Hypothesised increases in microbial activity can only exacerbate and increase concerns for altering the Arctic Carbon sink to further release unknown quantities of greenhouse gases. Therefore, there is need for more integrated research to fully understand and appreciate these high latitude ecosystems.
GIS Assessment of Glaciers and Climate Sensitivity, Southwestern Alaska
William F. Manley1
1Institute of Arctic and Alpine Research, University of Colorado, Campus Box 450, Boulder, CO, 80309-0450, USA, Phone 303-735-1300, Fax 303-492-6388, William.Manley [at] colorado.edu
Recent advances in Geographic Information Systems (GIS) make it possible to assemble large, empirical, multiparameter datasets that bear on environmental variation, process, and change. For example, GIS permits analysis of the extent, area-altitude relations, microclimatic, and major climatic relationships of all glaciers within a region. Complementary to laser-altimetry and field measurements of mass balance, this approach takes advantage of spatial, rather than temporal, variation to better understand glacier-climate relationships.
A case study for the Ahklun Mountains, southwestern Alaska, demonstrates the feasibility, resolution, and glacier-climate significance of the new approach. Data sources include high-resolution DEM's (grid-cell spacing of 62 m), gridded PRISM climate estimates, and digitized glacier outlines from 1:63,360 topographic maps (based on aerial photography from 1972–1973). Using raster GIS, 32 parameters were calculated for each of the 106 cirque and small valley glaciers in the Ahklun Mountains, including area, elevation, slope angle, aspect, curvature, potential insolation, backwall height, hypsometric Equilibrium Line Altitude (ELA; based on an Accumulation Area Ratio of 0.6), summer temperature, winter precipitation, and sensitivity to climate induced changes in ELA. The 106 cirque and small valley glaciers have a median size of 0.26 km2, a total area of 59.6 km2, and a statistically preferred aspect of 334°. Hypsometric ELA averages 929 m ± 127 m.
Ten percent of the ELA variation is explained by a trend surface dipping 5 m/km southwestward toward the Bering Sea as a moisture source. Inclusion of aspect, a basin coefficient, backwall height, distance from lakes, and upslope area in stepwise multiple regression brings explanation to a level of 52%, and highlights the importance of microclimatic/topographic controls on ELA and mass balance. Furthermore, 73% of ELA variation is explained by winter precipitation, summer temperature, aspect, and other microclimatic variables.
Sensitivity to a rise in ELA is estimated from area-altitude relationships. With an increase in ELA of only 50 m, accumulation areas would shift from ca. 60% of each glacier surface to only 28% on average, and total glacier area would with time decrease 40% to about 36 km2.
Errors for the parameters are insignificant in comparison with high local variability. Results include not only datasets, but the ability to draw meaningful relationships from spatial trends. The Ahklun glaciers will be strongly affected by climate-induced changes in accumulation or ablation.
An NSF-funded project was recently initiated to ascertain glacier-climate relationships across Alaska using GIS. This project will measure numerous parameters for all Alaskan glaciers across strong climatic and glaciodynamic gradients, will clarify climatic controls on mass balance, and will identify which glaciers are most sensitive to 21rst century climate change.
Interactions Between Arctic Terrestrial Ecosystems and the Climate System
A. David McGuire1
1Institute of Arctic Biology, University of Alaska Fairbanks, 216 Irving I Building, Fairbanks, AK, 99775, USA, Phone 907-474-6242, Fax 907-474-6716, ffadm [at] uaf.edu
Analyses by the Intergovernmental Panel on Climate Change (IPCC) project that the buildup of greenhouse gases in the atmosphere is likely to lead to increases in mean annual temperature of between 1.0 and 3.0 C° by 2100 with increases greater in high latitudes than in middle or low latitudes. There is evidence that warming is occurring in some high-latitude areas. Trends locally exceed 0.5° per decade, which are much larger than for the Northern Hemisphere as a whole, and are most pronounced in northwest North America. There is evidence that the warming in high latitude regions of North America may be affecting ecosystem function and structure of both tundra and boreal forest. These changes in high latitude terrestrial ecosystems have important consequences for the global climate system as well as for climate in arctic regions by influencing (a) water and energy exchange with the atmosphere, (b) the exchange of radiatively active gases with the atmosphere, and (c) the delivery of fresh water to the Arctic Ocean. As the responses of high latitude ecosystems to global change involve complex interactions among environmental variables that influence permafrost dynamics, vegetation dynamics, and disturbance regimes, there is a need for coordinated global change studies among high latitude regions. These studies should focus on improving understanding on how these responses influence climate dynamics, ecosystem dynamics, and large-scale hydrology throughout high latitude regions. It is important that scientific understanding from such studies be synthesized into integrated modeling efforts focused on representing the role of high latitude terrestrial ecosystems in the response of the climate system to global change. Integrated modeling efforts should focus on closing water, energy, and carbon budgets in retrospective studies at large scales in high latitude regions to help elucidate critical gaps in our understanding. Effective iteration between field- and model-oriented research is important for articulating the next steps that need to be taken in representing the role of high latitude terrestrial ecosystems in the climate system.
Teachers Experiencing Antarctica and the Arctic - TEA Bringing Research into Classrooms
Debra A. Meese1, Stephanie Shipp2, Clarice Yentsch3
1Snow and Ice Branch, Cold Regions Research and Engineering Laboratory, 72 Lyme Road, Hanover, NH, 03755, USA, Phone 603-646-4594, Fax 603-646-4644, dmeese [at] crrel.usace.army.mil
2Department of Geology and Geophysics, Rice University, MS-126, PO Box 1892, Houston, TX, 77251-1892, USA, Phone 713-348-2515, Fax 713-348-5214, shippst [at] ruf.rice.edu
3American Museum of Natural History, Central Park West at 79th Street, New York, NY, 10024-5192, USA, Phone 305-296-7174, cmyentsch [at] aol.com
The centerpiece of the Teachers Experiencing Antarctica and the Arctic (TEA) Program is a research experience in which a K-12 teacher participates in a polar field program. The TEA teacher works closely with scientists, participates in cutting-edge research, and is immersed in the process of science. Enveloping this field experience is a diversity of professional development opportunities through which TEA teachers increase content knowledge, enhance teaching skills, transfer the experience to the classroom, assume leadership roles, and collaborate with a network of researchers and education colleagues. TEA is a partnership between teachers, researchers, students, the school district, and the community.
TEA is sponsored by the Division of Elementary, Secondary, and Informal Education (ESIE) in the Directorate of Education and Human Resources (EHR) and the Office of Polar Programs (OPP) of the NSF and facilitated by Rice University, the Cold Regions Research and Enginerring Laboratoy (CRREL), and the American Museum of Natural History (AMNH).
The Sea Ice Biological Communities in Recent Environmental Changes in the Arctic
Igor A. Melnikov1, P. P. Shirshov2
1Institute of Oceanology, Russian Academy of Sciences, Nakhimovsky pr 36, Moscow, 117851, Russia, Phone +7-095/124-5996, Fax +7-095/124-5983, migor [at] online.ru
2Institute of Oceanology, Russian Academy of Sciences, Nakhimovsky pr 36, Moscow, AK, 117851, Russia
The warming trend is observed in the Northern and Southern hemispheres and it is more noticeable in the Arctic than elsewhere. The fundamental changes in the Arctic Ocean have led to a remarkable environmental changes in the Canadian Basin where there were indicated: (1) a noticeable shrinking of the sea-ice cover beginning from the regular satellite observations in 70th and (2) a rise in temperature and freshening of the Arctic surface waters associated with intensive melting of sea ice. As a consequence of these environmental changes within the air-ice-water system, the composition, structure and dynamic of sea ice and upper water biological communities have been also changed. Observations made in the Canadian Basin during the SHEBA (Surface Heat Budget in the Arctic Ocean) round-year experiment in 1997–1998 and during the Russian "Arctic-2000" expedition have shown that: (1) sea ice diatoms are very scarce by species and numbers; (2) fresh water green algae are dominated by numbers and distributed within the whole sea ice thickness; (3) invertebrate animals within the sea ice interior are not indicated; (4) invertebrate animals from the ice/water interface are scarce by species and numbers; (5) concentrations of chlorophyll and nutrients in the sea ice are significantly lower of the average concentrations measured before in this region for the same period of time. Remarkable accumulation of the organic mater within the sea ice interior was not indicated. Observed changes in the species composition and dynamic of the arctic sea ice ecosystem may be explained by the growing melting of the sea ice cover during the last decades. The main factors are: (1) drainage of fresh water throughout sea ice interior; (2) accumulation of fresh water beneath the ice; (3) formation of 2–3 m thick pycnocline at around 30–35 m. It seems that the recent water-ice system above pycnocline is more a freshwater-brackish system than the real marine system. It may suggest that dramatically changes within the sea ice environment can be considered as a result of global warming in the Arctic.
Visualizing Cetacean Habitats Offshore Northern Alaska
Sue E. Moore1, Jeremy R. Davies2
1National Marine Mammal Laboratory, NOAA/Alaska Fisheries Science Center, 7600 Sand Point Way NE, Seattle, WA, 98115, USA, Phone 206-526-4021, Fax 206-526-6615, sue.moore [at] noaa.gov
2National Marine Mammal Laboratory, National Oceanic and Atmospheric Administration (NOAA), 7600 Sand Point Way NE, Seattle, WA, 98115, USA, Phone 360/738-2844, Fax 240/269-7683, jeremy.davies [at] noaa.gov
Differences in cetacean habitats offshore northern Alaska have been described, based upon analyses of 10 years of aerial survey sighting data (Moore et al., 2000). One of the strongest differences described was that of bathymetric habitat selection by bowhead whales (Balaena mysticetus) and white whales (Delphinapterus leucas). In brief, filter feeding bowheads select the comparatively shallow waters of the continental shelf, while piscivorous white whales (also called belugas or belukhas) select continental slope waters. Although these differences can be clearly defined statistically, the power of a three dimensional display to illustrate the distribution of the two species in relation to bathymetry off the north shore of Alaska is striking (Figure 1). A further refinement to the depiction of bathymetric habitat separation is provided by a display of 75% fixed kermels around the distribution plots for each species (Figure 2). These figures provide dramatic portrayals of cetacean distribution, which invites collaboration with physical oceanographers and other marine biologists to further explore the habitat parameters important to Arctic cetaceans.
The USGS Yukon River Basin Water Quality Study
Gordon L. Nelson1, Timothy P. Brabets2, Richard P. Hooper3, Edward R. Landa4
1U.S. Geological Survey, 4230 University Dr., Suite 201, Anchorage, AK, 99508, USA, Phone 907/786-7111, glnelson [at] usgs.gov
2U.S. Geological Survey, 4230 University Dr., Suite 201, Anchorage, AK, 99508, USA, Phone 907/786-7105, tbrabets [at] usgs.gov
3U.S. Geological Survey, 10 Bearfoot Road , Northborough, MA, 01532, USA, Phone 508/490-5065, rphooper [at] usgs.gov
4U.S. Geological Survey, 430 National Center, Reston, VA, 20192, USA, Phone 703/648-5898, erlanda [at] usgs.gov
As part of its National Stream Quality Accounting Network (NASQAN) program, the USGS recently launched a five-year study of water quality in the Yukon River Basin of Alaska. A fixed station network consisting of five sites [Yukon River at Eagle, Porcupine River near Fort Yukon, Yukon River near Stevens Village, Tanana River at Nenana, and the Yukon River at Pilot Station] has been established. Sampling at the fixed sites began in October 2000. Water at these stations will be sampled approximately seven times per year (once under ice and six times during open water) and analyzed for major ions and trace elements, nutrients, organic carbon, and suspended sediment load. In addition to the baseline water quality measurements, topical studies focused on carbon cycling and climate change, mercury, potential microbial pathogen contamination, and contamination by persistent organic compounds will be undertaken. Opportunities for expanding the study and for collaborative research exist, and are encouraged at this early stage of program development.
The Arctic Oscillation as the Driver of Spring Warmings
James E. Overland1, Muyin Wang2, Nicholas A. Bond3
1Pacific Marine Environmental Laboratory, National Oceanic and Atmospheric Administration (NOAA), 7600 Sand Point Way NE, Seattle, WA, 98115, USA, Phone 206/526-6795, Fax 206/526-6485, overland [at] pmel.noaa.gov
2Joint Institute for the Study of the Atmosphere and Oceans, University of Washington, Box 354235, Seattle, WA, 98195, USA, Phone 206/526-4532, Fax 206/685-3397 , muyin [at] atmos.washington.edu
3JISAO, University of Washington, Box 354235, Seattle, WA, 98195-4235, USA, Phone 206/526-6459, Fax 206/685-3397, bond [at] pmel.noaa.gov
Conditions in the Arctic in the 1990s were substantially different than previous decades. Six of nine recent years (1990-1998) had major cold temperature anomalies in March in the stratosphere. The remaining three years had weaker cold anomalies. These cold anomalies are part of the Arctic Oscillation (AO), and models suggest that the persistence of the anomalies is driven by CO2 increases and ozone chemistry at cold temperatures in spring. Cold stratospheric anomalies are associated with warm tropospheric anomalies. Because the location of the stratospheric vortex varies from year to year, anomalous surface winds and warm temperatures occur over Alaska and Northwest Canada in years when the vortex was shifted to the western Arctic; i.e., in 1990, 1993, 1995 and 1997. Both the North Atlantic Oscillation (NAO) and the AO are influenced by increases in zonal winds from the polar vortex associated with the cold stratosphere; however, the NAO has winter variability reinforced by longitudinal sea-surface temperature gradients, while the Arctic proper has a hemispheric component driven by the stratospheric change in late winter. Although the stratospheric/tropospheric connection decouples in May, earlier snow and ice melt in late spring in the western Arctic may precondition summer and fall conditions through albedo and cloud/radiative feedbacks.
Erosion of Community Stability by Large-Scale Climatic Change
Eric Post1
1Department of Biology, Penn State University, 208 Mueller Lab, University Park, PA, 16802, USA, Phone 814-865-1556, Fax 814-865-9131, esp10 [at] psu.edu
Large-scale climatic change may threaten the stability of biological communities because, according to ecological theory, stability is only possible in stochastic environments if there is sufficiently strong self-regulation at one or more trophic levels. Using long-term data from Isle Royale, USA, on the dynamics at three trophic levels (wolves, moose, and balsam fir), we developed and tested a model of community dynamics and stability in a changing climate. This analysis revealed that large-scale climatic variability influenced dynamics directly at all three trophic levels and indirectly, through predation, at the top two levels. The community matrix indicated that the community was stable. Stability eroded, however, when the system was modeled with either the direct or indirect influences of climate held constant. The loss of community stability was traceable to the deterioration of self-regulation at the top and bottom trophic levels as interactions with the middle trophic level intensified. These results suggest that sudden climatic changes, such as the periodic attenuation of interannual variability in a warmer climate, have the potential to alter the stability of biological communities.
Reconstructing Late Holocene Environments from Laminated Sediments, Cape Hurd Lake, Devon Island, Nunavut, Canada
Michael J. Retelle1, Mary Katherine Fasy2, Forrest Janukajtis3, Douglas R. Hardy4
1Department of Geology, Bates College, 44 Campus Avenue, Carnegie Science Center, Lewiston, ME, 04240, USA, Phone 207-786-6155, Fax 207-786-8334, mretelle [at] bates.edu
2DEpartment of Geology, Bates College, USA
3Department of Geology, Bates College, USA
4Department of Geosciences, University of Massachusetts, MA
In light of recent projected impacts of climate change in the arctic regions, the need has arisen for development of a network of continuous and high resolution archives of past climate that provide records that demonstrate the range and spatial variability of climate change. Such archives include ice cores, tree rings (from at or below treeline) and continuous marine and lacustrine sediment records obtained from cores. Ideally lacustrine and marine sediments are annually laminated and may yield annual or even seasonal signals of climatic, hydrologic, limnologic and biogenic processes recorded in composition of the laminated components. Varved sediments have been found in several settings in the high arctic including proglacial lakes and coastal isolation basins. In these isostatically controlled meromictic lakes and density-stratified coastal inlets, optimal laminated sediment preservation occurs due to density-stratification of the basin with anoxic and saline bottom water trapped at depth behind an emerging bedrock sill. In these isolated basins, stagnant bottom water excludes occupation and burrowing by bottom-dwelling fauna.
This poster focuses on Cape Hurd Lake, a coastal basin on southwestern Devon Island (74°34' N, 89°38' W) . Cape Hurd Lake is presently at sea level and is connected to adjacent marine waters by a narrow, shallow channel incised through a sandy and gravelly emergent spit which served as the threshold or sill that isolated the basin from the sea. The watershed of the lake is 47 km2 and has two main inlet streams which feed contribute runoff from watershed snowpack and glacier meltwater from an adjacent plateau ice cap. The lake basin is steep sided with a flat-floored, central basin with a maximum depth of 55 m. Prior to runoff in May to early June, 1999 and 2000, ice thickness ranged from 1.5 to 2.0 meters. The water column is hypersaline (43ppt) below 35 meters, and is overlain by a cap of less saline marine water. In late summer, the basin is generally ice-free and seawater enters the basin through the narrow outlet and circulates in the epilminion.
Climatic measurements are recorded in the watershed with an automated weather station situated on the edge of the adjacent plateau north of the lake. In addition, a programmable digital camera linked to the weather station records and stores time-lapse photographs of the watershed allowing for monitoring of hourly sky conditions, snowpack, runoff, lake ice extent, and sea ice in the channel beyond the lake. In the lake, ice thickness, secchi depth are monitored, and water column conditions are measured using a CTD instrument that records temperature, salinity, dissolved oxygen and light transmission. Sediment traps with funnels were deployed at various sites in the lake to determine seasonal sediment flux. A submersible video camera was used to reveal the extent of ice algae growing on the underside of the ice cover and the nature of the basin floor.
Sediment cores up to 3 meters in length were recovered in several transects across the basin. The sediment core records have been organized into litho- and biostratigraphic zones based on sediment textures, lamination type and biotic components:
Zone 1: The base of the core is comprised of massive mud interrupted by thin, disturbed biogenic/terrestrial laminations. The massive mud contains marine macro and microfossils and is a result of deposition in an open marine inlet prior to the onset of anoxia in the basin.
Zone 2: A thick (2.5 m) sequence of laminated sediments overlies the massive mud. The bottom of the laminated sequence begins with diffuse laminae overlain by biogenic-terrestrial couplets comprised of diatoms and cysts with fine silty terrigenous mud cap. The fine couplets reflect onset of anoxia in the basin with seasonal terrigenous sediment influx coupled with marine biogenic productivity and preservation of the organic matter on the basin floor. The laminated sediments are interbedded with three layers (7 to 10 cm thick) of massive, bioturbated mud containing forams, diatoms and fecal pellets. This zone likely reflects breaching of the newly emerged bedrock sill and re-oxygenation of the basin.
Zone 3: Laminated sediments are variable in texture and composition. The zone is dominated by terrestrial laminae and accompanied by an increase in grain size. Diatoms and organic matter are rare in this zone.
Zone 4: Zone is variable in grain size as a result of the frequent occurance of turbidity current deposition. Composition of the lighter layers in the zone progressively grades from Si-rich to Ca-rich reflecting decrease in diatomaceous material and increase in detrital carbonate.
Zone 5 Laminae gradually become thinner and finer until the laminations become diffuse. Loss-on-ignition data and EDS maps indicate an increase in organic material.
Zone 6: The uppermost sediments in the cores are characterized by an increase in grain size and presence of diatoms. Numerous fining-upward turbidite sequences likely reflect delta instability due to increased terrestrial sediment flux, possibly due to increased glacier runoff.
Land Cover Change on the Seward Peninsula: The Use of Remote Sensing to Evaluate the Potential Influences of Climate Warming on Historical Vegetation Dynamics
Cherie S. Silapaswan1, David L. Verbyla2, A. D. McGuire3
1Department of Biology and Wildlife, University of Alaska Fairbanks, Fairbanks, AK, 99775, USA, ftcss1 [at] uaf.edu
2Department of Forest Sciences, University of Alaska Fairbanks, Fairbanks, AK, 99775, USA, Phone 907/474-5553, Fax 907/474-6184
3U. S. Geological Survey, Alaska Cooperative Fish and Wildlife Research Unit, University of Alaska Fairbanks, Fairbanks, AK, 99775, USA, Phone 907/474-6242, Fax 907/474-6716, ffadm [at] uaf.edu
Vegetation on the Seward Peninsula, Alaska, which is characterized by transitions from tundra to boreal forest, may be sensitive to the influences of climate change on disturbance and species composition. To determine the ability to detect decadal-scale structural changes in vegetation, Change Vector Analysis (CVA) techniques were evaluated for Landsat TM imagery of the Seward Peninsula. Scenes were geographically corrected to sub-pixel accuracy and then radiometrically rectified. The CVA detected vegetation change on more than 50% of the burned region on TM imagery for up to nine years following fire. Between the 1986 and 1992 satellite scenes, the CVA detected changes in direction and magnitude of the two indices (TM Band4/TM Band 3, TM Band 5). Overall, approximately 759,610 ha changed to a class with a more developed canopy and only 268,132 ha changed to a class with a less developed canopy. CVA results and photo interpretation together show that shrub advance is approximately 100 m in valleys north of the Bendeleben Mountains and that shrubs have increased along riverbed bottoms. Thus, the change detection analysis based on the unsupervised classification indicates that land-cover change on the Seward Peninsula was predominantly in the direction of increased shrubbiness. Taken together, our comparison of CVA results, unsupervised classification results, and visual interpretation of aerial photographs suggests that shrub cover may be increasing on the Seward Peninsula, which is consistent with results from experimental warming in tundra. The use of both CVA and unsupervised classification together provided a more powerful interpretation of change than either method alone in transitional regions between tundra and boreal forest.
Polar Cap Disturbances: Mesosphere and Thermosphere - Ionosphere Response to Solar-Terrestrial Interactions
Gulamabas G. Sivjee1, D. J. McEwan2
1Department of Physical Sciences, Embry Riddle Aeronautical University, 600 S Clyde Morris Blvd, Daytona Beach, FL, 32114, USA, Phone 386-226-6711, Fax 386-226-6621, sivjee [at] db.erau.edu
2Department of Physics and Engineering Physics, University of Saskatchewan, 116 Science Place, Saskatoon, S7N 5E2, Canada, Phone 306-966-6440, Fax 306-966-6400
The Polar Cap is the Upper-Atmosphere cum Magnetosphere region which is enclosed by the poleward boundary of the Auroral Oval and is threaded by open geomagnetic field lines. In this region, there is normally a steady precipitation (Polar Drizzle) of low energy (~ 100eV) electrons which excite optical emissions from the ionosphere. At times, enhanced ionization patches are formed near the Dayside Cusp region which drift across the Polar Cap towards the Night Sector of the Auroral Oval. Discrete auroral arcs and auroras formed during Solar Magnetic Cloud (SMC)/Coronal Mass Ejection (CME) events are also observed in the Polar Cap. Spectrophotometric observations of all these Polar Cap phenomena from the Arctic stations in Longyearbyen (79° N), Svalbard, Eureka (80° N) and Resolute Bay (76° N), Canada as well Sondrestromfjord (67° N), Greenland provide a measure of the average energy as well as energy flux of the electrons precipitating in the Polar Cap region during these disturbances. Such measurements also point to the Planetary, Tidal and Gravity Wave Modulations of the Polar Mesosphere-Lower Thermosphere (MLT) during six months long dark polar winters. Most of the Polar Cap MLT air density and temperature modulations appear to represent the effects of zonally symmetric tides whose Hough functions peak in the Polar region. MLT cooling during Stratspheric Warming events and their relation to Polar Vortex and associated Gravity wave activities are also observed at the Polar Cap sites. Results of optical remote sensing of these Polar Cap phenomena from the four Arctic stations are discussed.
Geographic Information Infrastructures: Applications for Science and Policy
Mark Sorensen1
1Geographic Planning Collaborative Inc (GPC), PO Box 1179, Running Springs, CA, 92382, USA, Phone 909/867-7628, Fax 909/867-5310, gpci [at] aol.com
Anthropogenic signals recorded in an ice core from Eclipse Icefield, Yukon Territory, Canada
Cameron P. Wake1, Kaplan Yalcin2, Deana Aulisio3
1Climate Change Research Center, EOS, University of New Hampshire, Morse Hall, Durham, NJ, 03824, USA, Phone 603-862-2329, Fax 603-862-2124, cameron.wake [at] unh.edu
2Climate Change Research Center, EOS, University of New Hampshire, Morse Hall, Durham, NH, 03824, USA
3Climate Change Research Center, EOS, University of New Hampshire, Morse Hall, Durham, NH, 03824, USA
Arctic Sea Ice Oscillation: Regional and Seasonal Perspectives
Jia Wang1, Moto Ikeda2
1International Arctic Research Center-Frontier Research Syste, University of Alaska Fairbanks, 930 Koyukuk Dr., Fairbanks, AK, 99775-7335, USA, Phone 907/474-2685, jwang [at] iarc.uaf.edu
2Graduate School of Environmental Earth Science, Hokkaido University, 060, Japan
Variability of the sea-ice cover (extent) in the Northern Hemisphere (Arctic and subpolar regions) associated with AO (Arctic Oscillation) is investigated using historical data from 1901 to 1997. A principal component analysis (empirical orthogonal functions, EOFs) was applied to sea ice area (SIA) anomalies for the period 1953-95. The leading EOF mode for the SIA anomaly shows an in-phase fluctuation in response to AO and is named ASIO (Arctic sea ice oscillation). Arctic sea ice experiences seasonal variations of different types in timing and magnitude. Four types of seasonal variations are identified in the Arctic sea ice, superimposed on long-term interannual to decadal variability. Consistent with the total Arctic SIA anomaly, eight regional SIA anomalies have shown significant in-phase decrease (downward trend) since 1970, possibly part of a very long-term (century) cycle. Thus, it is recommended that SIA anomalies in the sensitive seasons be used to better capture interannual, interdecadal, and longer (century) variability. Major decadal and interdecadal time scales of SIA anomalies are found 12-14 and 17-20 years. In the Sea of Okhotsk, a century time scale is evident. The reduction rate (negative trend) of the total Arctic sea ice cover in the last three decades is -4.5% per decade with the summer rate being the highest (-10.2% per decade). The contribution to this total reduction varies from region to region, among which sea-ice cover in the Greenland and Norwegian Seas experiences the highest reduction rate of -20.2% per decade.
The David Brown Book Company Book Exhibit
Amanda M Young1
1The David Brown Book Company, 28 Main Street, Oakville, CT, 06779, USA, Phone 860-945-9329, Fax 860-945-9468, amanda.young [at] snet.net
The David Brown Book Company will be representing Aarhus University Press and University of Iceland Press (available for the first time in North America.) Our display will include books on anthropology, archaeology, politics and economics in arctic regions.
Please come browse our selection and take advantage of the 20% conference discount.